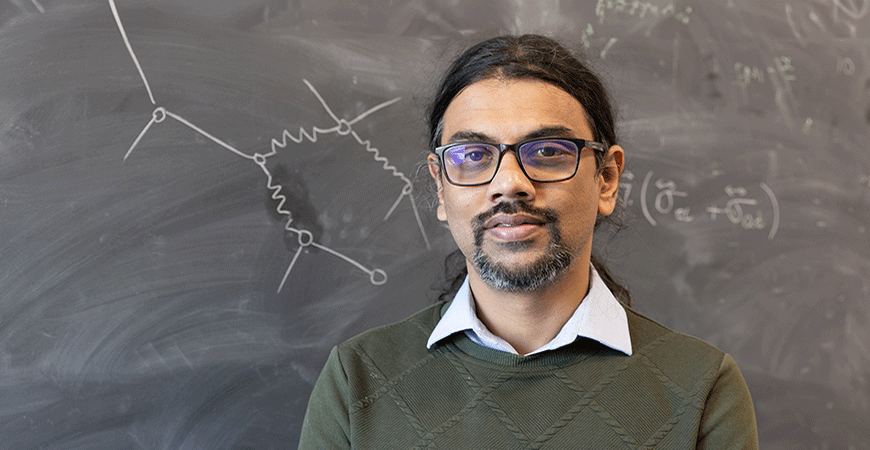
A group of researchers, including physicists from UC Merced, has made discoveries about the mechanics of blood clots that could help in the development of biomaterials for tissue engineering and improved surgical adhesives.
Many materials around us, including those in cells and tissues, are made of networks of elastic fibers that resist deformation. These biological fibrous networks determine the fine balance between health and disease.
One such branched network, comprising fibrin fibers enmeshed with blood cells, is the blood clot. To stop bleeding, a clot needs to form (and deform) to the right size and shape and be strong enough to withstand the forces of blood flow.
If scientists can understand the mechanical behavior of blood clots, developing methods for controlling them — and engineered biomaterials like them — would be possible.
“Fibrin gels are used as surgical adhesives and sealants because they are biocompatible. Our work implies that the mechanical property of the sealants can be tailored to suit the application,” explained Professor Kinjal Dasbiswas, who researches soft condensed matter as a member of the Department of Physics at UC Merced.
“For instance, different stages of wound healing may require different mechanical properties and responses to stimuli, such as closing the wound, allowing immune cells to infiltrate, new cells to regenerate and promoting new tissue growth,” Dasbiswas and his collaborators said. “Our study shows that by altering the number of molecular crosslinks, we can control the mechanical behavior of the material. This provides an opportunity to develop new fibrin-based biomaterials by altering the levels of crosslinking.”
To study the mechanical behavior of clots, Andrei Zakharov, a former postdoctoral researcher with Dasbiswas’s research group, developed a network model of connected spring-like bonds that represent the fibrin network.
Two surprising observations immediately stood out when the fibers were allowed to bend and buckle easily in the network simulations. First, under increasing external load, the network of fibers becomes softer before it begins to stiffen. Second, the easily bent fibers transmit less force, leading to less overall platelet-driven contraction of the network.
The study showed, both with computer simulations of an elastic network model and experiments on blood clots, that the clot’s proper biological function relies on the ability of individual fibers to bend and buckle under mechanical loads.
To understand clots’ mechanical responses under different conditions, the bonds underwent various types of deformation, such as stretching, compression, bending and buckling.
“The deformations could be created either by external load or internal platelet-generated forces,” Dasbiswas said. “It is typically much easier to bend or buckle a thin fiber than to stretch it. Think of your computer or TV power cable, which you can bend much more easily than stretch.”
Dasbiswas, bioengineering Professor Arvind Gopinath and professors John Lee and Anand Ramasubramanian at San Jose State University together hypothesized that inhibiting factor XIII — a blood component that facilitates crosslinking of individual fibrin protofibrils into fibers — would reduce the bending stiffness of fibrin fibers.
To test this, Myra Awan, a master’s student in Ramasubramanian’s lab, measured the stiffness of clots under increasing shear stress. She showed that, indeed, the crosslinker-inhibited clots softened before they stiffened, as Andrei’s model predicted.
“Think of what happens when we pull on a crumpled piece of paper or cloth. It first expands easily but then becomes difficult to stretch as you pull out the slack,” Dasbiswas said. “This is an example of materials becoming stiffer as you deform them. We, however, show that when clots with reduced crosslinkers are progressively deformed, they first become soft before stiffening.
“Because most biopolymer networks stiffen under shear, this was surprising and to our knowledge, the first demonstration of this additional softening regime in a biomaterial,” Dasbiswas said. “This work is also a great example of how physics-based modeling and careful experimentation, along with collaboration, can successfully reveal aspects of the complex behavior of biological materials.”